Assistant professor in chemical engineering awarded NSF grant to design stronger, more durable membranes to be used during reverse osmosis
Published: Aug 9, 2024 1:00 PM
By Joe McAdory
Americans consume more than 7.5 million gallons of bottled water annually, according to the Food and Drug Administration. However, obstacles persist.
“With population growth comes increased pollution, which reduces freshwater sources,” said Cassandra Porter, assistant professor in the Department of Chemical Engineering. “That said, we have begun using non-traditional sources, such as seawater. It’s not an infinite source, but it’s much larger.”
The most effective means to desalinate seawater is reverse osmosis (RO) using polyamide membranes.
Why are RO polyamide membranes so effective in removing salt from water? Why do they often develop biofilms? Why do their molecular structures disintegrate during chlorination? How can they be made better?
Porter intends to find out.
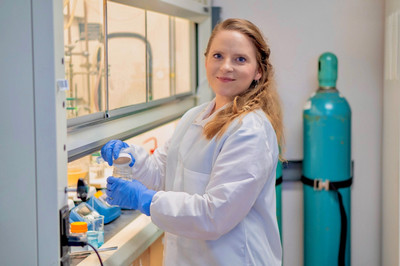
Her proposal, “Polyamide Brush Active-Layer Membranes for Fundamental Understanding of Structure-Function Relationships in Thin-Film Composite Reverse Osmosis Membranes,” earned a three-year, $303,707 National Science Foundation grant to develop a better understanding of RO polyamide membranes and create a new membrane that resists chlorine damage without minimizing performance.
Porter will investigate the hypothesis that weak intermolecular interactions play key roles in the performance of polyamide RO membranes.
“What we're doing is not focusing on the entire process of desalinization but getting new membranes in my laboratory to better desalinate contaminated water that the current reverse osmosis membranes cannot handle,” Porter said. “These contaminants include organics, compounds that infiltrate current commercial reverse osmosis membranes more easily.”
The effects of crosslinking, amide, benzyl and carboxyl density will be explored by producing a highly tailorable, novel polyamide membrane built with a dense layer of brush polymers – the brush-active layer membrane.
“Most membranes are cast top down," Porter said. "Typically, you mix the viscous polymer dopant, smear it on a glass plate, gel it and it develops pores through phase separation. For polyamide RO membranes, two reagents react at the interface of water and organic phases, polymerizing rapidly with little control.
“What’s unique about brush active-layer membranes is they grow bottom-up using surface-initiated atom-transfer radical polymerization. Our bodies are grown bottom-up. There's a blueprint, DNA, and it imparts into the human body any function you want. The idea is that if we grow the membrane bottom-up, and we can control every molecule that goes into it, we will generate a superior performance.”
Membrane performance will be assessed by measuring salt and water permeability and molecular cutoff while targeting selectivity comparable with commercial RO membranes. The control over membrane thickness and grafting density will be exploited to hone membrane transport models.
“We'll be able to produce membranes that are more resistant to cleaning, have greater selectivity as far as being able to reject more salt, and might even separate out key elements that are found in water, like lithium,” Porter said. “The platform hopefully will allow us to, by changing various functional groups, target all of these different separations that we're not capable of doing right now.”
Media Contact: , jem0040@auburn.edu, 334.844.3447
Cassandra Porter earned a three-year, $303,707 grant from the National Science Foundation.